![]() |
The aerospace industry has used aluminum and its alloys for parts and skins for decades. Today it is the most common material used in the industry; used in the manufacture of advanced commercial aircraft such as the Boeing 777 and Airbus 380, and military aircraft such as the Boeing UCAV or the Boeing F/A-18 E/F (Figure 1). This is because aluminum, heat-treated to relatively high strengths, machines and forms easily to complex shapes and low weight.
Commonly employed for wrought products with thicknesses of 0.6mm to 250mm are aluminum alloys in the 7000 series. Used extensively for stringers and other structural requirements are extrusions of 7075 and 7050 alloys, as well as some 2024 and 2014 alloys. Clad and non-clad sheets of 2024, 7475, and 7075 find use for wing and fuselage skins, bulkheads, and other structural elements. Commonly used to fabricate large ribs and bulkheads, particularly in military aircraft are large forgings of 7050 and 7040.
To minimize the cost of large forgings, many manufacturers are looking into mill heat-treated 7050 and 7040 plate. This eliminates heat treatment after machining, allowing machining of parts to net shape. Unfortunately, there is a significant property variation through the thickness of thick plate. Heat-treating of aluminum requires stringent controls. Development of a series of specifications, by airframe manufacturers, suppliers, and heat-treaters is to achieve repeatable results, and to provide a quality product. The most widely used specification is AMS-2770 “Heat Treatment of Aluminum Parts.”
Aluminum Heat Treating
Classification of aluminum alloys are as either heat-treatable or not heat-treatable, depending on whether the alloy responds to precipitation hardening. Showing greater solubility at elevated temperatures, than when they are at room temperature, are heat-treatable alloy systems – such as 7000-, 6000-, and 2000-series materials. Use of controlled precipitation of the solute at room temperature (natural aging) or at elevated temperatures (artificial aging) is to develop the optimized mechanical and corrosion properties of these alloys.
Quenching
The nucleation theory applied to diffusion controlled solid-state reactions helps understand heterogeneous precipitation during quenching. The kinetics of heterogeneous precipitation occurring during quenching is dependent on the degree of solute supersaturation and the diffusion rate, as a function of temperature. Therefore, as an alloy is quenched, there is greater supersaturation (assuming no solute precipitates). However, the diffusion rate increases as a function of temperature. The diffusion rate is greatest at elevated temperature. When either the supersaturation or the diffusion rate is low, the precipitation rate is low.
At intermediate temperatures, supersaturation and diffusion rate are relatively high. Therefore, the heterogeneous precipitation rate is the greatest at intermediate temperatures (Figure 3). The quench rate governs the amount of time spent in this critical temperature range. The amount of precipitation occurring during quenching reduces the amount of subsequent hardening possible. This is because as solute precipitates from solution during quenching, it is unavailable for any further precipitation reactions, with resultant loss of strength and ductility.
The Time-Temperature-Tensile Property curve for aluminum shows that the critical temperature range for 75S is 290°C to 400°C. This is similar to the critical temperature range found for Al-Zn-Mg-Cu alloys. Obtaining maximum strength and corrosion resistance was at quench rates exceeding 100°C/sec.
Natural Aging
Some heat-treatable alloys, especially 2000 series alloys, harden appreciably at room temperature to produce the useful tempers T3 and T4. These alloys exhibit high ratios of ultimate tensile strength/yield strength. They also have excellent fatigue and fracture toughness properties. The increase in properties occurs by the rapid formation of GP (Guinier-Preston) Zones from the supersaturated solid solution and from quenched-in vacancies. Strength increases rapidly, with properties becoming stable after approximately 96 hours.
The Al-Zn-Mg-Cu and Al-Mg-Cu alloys (7000 and 6000), harden by the same mechanism of GP Zone formation. However, the properties from natural aging are less stable. In 7000-series alloys, nearly full suppression of natural aging is possible when holding at -40°C, allowing the performance of forming without property changes.
Artificial Aging
After quenching, most aerospace aluminum alloys are artificially aged. This achieves greater strength. In general, the sequence of precipitation occurs by clustering of vacancies; formation of GP Zones; nucleation of a coherent precipitate; precipitation of an incoherent precipitate; and finally the coarsening of the precipitates.
Precipitation hardening is the mechanism where the hardness, yield strength, and ultimate strength increase dramatically with time at a constant temperature (the aging temperature) after rapidly cooling from a much higher temperature (solution heat-treat temperature). The rapid quenching results in a supersaturated solid solution, providing the driving force for precipitation.
Precipitation hardening (aging) involves heating the alloyed aluminum to a temperature in the 200°F to 450°F range. At this temperature, the supersaturated solid solution created by quenching from the solution, heat-treating temperature, begins to decompose. Initially there is a clustering of solute atoms near vacancies. Once sufficient atoms diffuse to these initial vacancy clusters, coherent precipitates form.
Because the clusters of solute atoms have a mismatch to the aluminum matrix, a strain field surrounds the solute clusters. As more solute diffuses to the clusters, eventually the matrix can no longer accommodate the matrix mismatch, and semi-coherent precipitate forms, which is the peak aged or highest strength achievable.
Finally, after the semi-coherent precipitate grows to a large enough size, the matrix can no longer support the crystallographic mismatch, and the equilibrium precipitate forms. This is shown in Figure 8 for 7050, showing the structural changes that occur from the formation of GP Zones to the final over-aged equilibrium precipitate.
Heating the quenched material in the range of 95°C to 205°C accelerates precipitation in heat-treatable alloys. In general, the increase in yield strength that occurs during artificial aging increases faster than the ultimate tensile strength. This means that the alloys lose ductility and toughness. T6 properties are higher than T4 properties, but ductility reduces.
Over-aging decreases the tensile strength, while increasing the resistance to Stress-Corrosion-Cracking. In addition, it enhances the resistance to fatigue crack growth and imparts dimensional stability of the part.
Developed for all the most common alloys are precipitation hardening curves. Generally, the higher the aging temperature, the shorter the time required for attaining maximum properties. When using high aging temperatures reaching properties, occur rapidly. For this reason, aging temperatures are usually lower to assure that the entire load obtains the required aging temperature without risk of reduced properties caused by over-aging of the fastest rising aluminum.
Distortion Occurs
Distortion is the most common problem occurring during aluminum heat treatment. The cause of distortion during quenching is from differential thermal strain development during quenching. Development of these thermal strains can be center-to-surface or surface-to-surface. Occurrence of this differential cooling can be due to by large quench rates, so that the center cools much slower than the surface or by non-uniform heat transfer across the surface of the part.
Aluminum requires high quench rates to prevent premature heterogeneous precipitation occurring during quenching, and to maintain supersaturation of the solute. The coefficient of linear expansion of aluminum is approximately twice that of steel (2.38 x 10-5mm/mm compared to 1.12 x 10-5mm/mm). This means that thermal gradients in aluminum are twice as likely to cause distortion and residual stress as steel for the same thermal gradient. Additionally, aluminum is much less strong than steel at solution heat-treating temperatures, and more prone to damage.
This causes much greater changes in length or volume as a function of temperature, increasing the probability that distortion will occur. Racking of the parts is critical. The parts require full support, with the load covering a large area, since the creep strength of aluminum is poor. Loosely wiring parts will prevent the parts from hitting each other during solution heat treatment.
Parts should enter the quenchant aerodynamically to avoid distorting the part before it even enters the quenchant. This is because the aluminum is soft at solution heat-treating temperatures, and the hydrostatic force of entering the water can result in distortion. It should enter the quenchant smoothly – not slap the quenchant – so there is low hydrostatic forces on the part.
The use of polyalkylene glycol (PAG) polymer quenchants control and minimize distortion. Governed by AMS 3025, these quenchants are either Type I or Type II. Type I quenchants are single polyalkylene glycol polymers, while Type II quenchants are multiple molecular weight olyalkylene glycol polymers; each offering different benefits.
Because of the higher molecular weight of the Type II PAG quenchants, lower concentrations are useable. However, Type II polymers have a lower cloud point temperature, which can cause higher drag-out if removal of parts from the quenchant before they reach the quenchant temperature (typically 80ºF to 100ºF) occurs.
Polyalkylene glycols exhibit inverse solubility in water; completely soluble at room temperature, but insoluble at elevated temperatures. Solubility temperatures range from 60ºC to 90ºC, depending on the molecular weight and structure of the polymer.
This phenomenon of inverse solubility modifies the conventional three-stage quenching mechanism, providing great flexibility to control the cooling rate. At first immersion of a component, the solution in the immediate vicinity of the metal surface heats to above the inverse solubility temperature. The polymer becomes insoluble and a uniform polymer-rich film encapsulates the surface of the part. Heat transfer occurs by conduction, with the result of fast and uniform heat transfer on all surfaces of the part. The stability and the duration of this polymer film are dependent on the temperature, concentration, and amount of agitation present.
The stable, polymer-rich film eventually uniformly collapses, and cool quenchant comes into contact with the hot metal surface. When the surface temperatures fall below the reverse solubility temperature, the polymer dissolves, forming a homogeneous solution again [Above right].
Altering of the cooling rate of these polymers is possible by changing the concentration, quenchant temperature, and the amount of agitation. Typically, change of the concentration fixes the agitation. The polymer’s concentration influences the film thickness, and as the concentration increases, the maximum cooling rate decreases.
Agitation has an important effect on polymer quenching. It ensures uniform temperature distribution around the part and affects the quench rate. As the severity of agitation increases, the duration of the polymer-rich phase decreases and eventually disappears, and the maximum rate of cooling increases.
Conclusion
While aluminum alloys have a long history of use in aerospace applications, there are countless additional places for use of these alloys to save weight without sacrificing strength. Properly heat-treating aluminum alloys will improve performance properties, expanding applications for aluminum and improving its performance in existing applications.
Houghton Int'l Inc.
Valley Forge, PA
houghtonintl.com
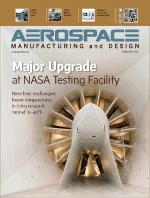
Explore the April May 2012 Issue
Check out more from this issue and find your next story to read.
Latest from Aerospace Manufacturing and Design
- Revitalizing the Defense Maritime Industrial Base with Blue Forge Alliance
- Safran Defense & Space opens US defense HQ
- Two miniature absolute encoders join US Digital’s lineup
- Lockheed Martin completes Orion for Artemis II
- Cylinder CMMs for complex symmetrical workpieces
- University of Oklahoma research fuels UAS development
- Motorized vision measuring system
- Everyone's talking tariffs