It is Friday afternoon and you, the engineer on duty in a manufacturing plant, get a call from someone on the factory floor: A heavy tool has been dropped on a composite structure that now must be assessed for damage. When you are face to face with the damaged part, the first thing you must decide is whether it is repairable, and if so, what type of repair is needed, or will the part need to be replaced. Let us look through the choices, which may differ from what a field repair requires.
Repair of advanced composite structures has been successfully accomplished for many decades. However, the usual context for such repair has been in an operational environment, and most of the work in this area focuses on the repair of damaged aerospace and marine structures. Damage in this context is primarily caused by impacts (either accidental or combat damage), lightning strikes, or overheat damage (exhaust areas, fires, etc.)
While lightning strikes are not usual in a manufacturing context, damage often results from accidental impacts, but also can have other causes not commonly seen in an operational environment. Examples are misdrilled holes, mistakes in trimming cured parts, adhesive-bonding errors, vacuum bagging errors leading to bridging or porosity, resin rich or resin starved areas, ply orientation, and other layup mistakes.
Repairs are easy and quick; other times they can be so difficult and expensive that it is better to scrap the component completely and build another. However, in many cases you will have to make a difficult decision for parts costing hundreds of thousands of dollars. It is also possible that the repair itself can cause further damage, and, in severe cases, can force you to scrap the part you are trying to save.
In an ideal world, careful control of the materials and processes used during manufacturing would prevent mistakes. However, as human labor increases in these processes, so does the risk of mistakes. Although a lot of effort goes into preventing errors in the automated manufacturing of large structures, such as transport category aircraft fuselage barrels, they can still be damaged when touched labor is involved. The manufacturing of smaller components, especially in low-volume aerospace manufacturing, uses only touch labor by skill technicians, because automated manufacturing these complex parts is too expensive. That is why comprehensive, hands-on training for technicians is vital to reducing errors and their associated high costs.
Repairing some manufacturing mistakes can be easy. For example, if a component is accidentally trimmed slightly oversize on one edge after the cure, then it is not difficult to trim it a bit more to the proper size. However, if the same component is trimmed undersize, then the repair is significantly more difficult. If a hole is drilled slightly off center, then the repair to fill the hole and redrill it in the correct location is more difficult than one might think. In a heavily loaded lightweight structure, one cannot simply fill the hole with putty and redrill it.
In any structural repair of flight-critical carbon-fiber epoxy aerospace structures, there are several goals. One is trying to match, but not to necessarily exceed, the original undamaged component’s strength (tensile and compressive), bending stiffness, which is a very different consideration from strength, and weight. On an aerodynamic surface, one is also trying to match the original outer mold line contour in the repaired area. There can be other considerations as well, such as radar signature, cosmetic appearance, etc.
To match original properties, one choice is to take the damaged component and throw it away, and replace it with a new component. Sometimes this may be the best solution, but economic and time considerations likely will rule out this approach.
In an actual structural composite repair, unfortunately, it is not possible to match exactly the strength, stiffness, and weight, even in theory. There is always a compromise somewhere. Since we are not performing thick structural repairs in these examples, we will most likely be performing a bonded repair, as opposed to a mechanically fastened repair, because thin laminates do not transfer loads well through mechanical-fasteners. Since the loads are being transferred through a new bondline, which was not there in the original structure, the tensile strength and compressive strength may decrease, since there are not continuous fibers carrying loads through the bondline. Adhesive bonds are by far the strongest in shear loading, so the bondline has to be designed to transfer the structural loads in shear.
To match the tensile and compressive strength of the undamaged component, the typical practice is to add one or more extra repair plies. For example, to repair a four-ply face sheet in a sandwich structure, one would likely do a five-ply repair. The extra ply can get us back to the original tensile and compressive strength, but due to the thickness, the bending stiffness would be higher than original. The extra thickness would also not create a perfectly flush aerodynamic surface. The extra stiffness and thickness would likely not matter on an interior component, or perhaps on the inside of a landing gear door, for example. However, during the repair of a helicopter rotor blade, these would be major concerns. Therefore, we need to distinguish between strength-critical repairs and stiffness-critical repairs in the engineering design of the repair.
In order to get good shear load transfer in a bonded repair, typically a scarfed repair is used. In such a repair, the damaged composites are scarfed, also called taper sanding, out from the original damaged area at a taper angle which may vary from as low as 25:1 in a lightly loaded structure up to as much as 100:1 in a heavily loaded area. So if the original structural damage is a small area of impact damage through a four-ply face sheet on a sandwich panel, which is made of woven cloth 0.010" thick per ply, then the 0.040" thick structure, at a 50:1 scarf angle, would be taper-sanded back 2" all around the damaged area.
If the original impact damage area was a puncture 1" in diameter, then the outer diameter of the scarfed area would be 5". Replacing the underlying sandwich core would be done first in the damaged area. Next is the bonding of the adhesive and repair plies to the surface. A typical sequence from the bottom up would be a thin layer of adhesive over the entire repair surface, a 1" diameter filler ply to cover the core, and then 2", 3", 4", and 5" diameter repair plies, which would be oriented to match the ply orientation of their matching underlying plies. Then a 6" diameter extra repair ply would be added, and its ply orientation would match the orientation of the original structural outer ply. Adhesive and plies are cured in place, often using a heat blanket, vacuum bag, and hot bonder.
In the repair of a poorly-drilled or slightly misplaced fastener hole, there may be special oversized fasteners that would allow the hole to be drilled out slightly and used as is. If the problem is too serious for this solution, then a scarfed repair on the edgeband may be necessary. Ideally, depending on the access, a scarf on both sides of the damaged area would be preferred, meeting in a tapered edge. Repair plies would be laid up with adhesive on both sides, then more would be added, and the repair would be cured. If two-sided access is too difficult, then the repair can be done from one side, using no backside access techniques.
Properly done, these repairs are permanent structural repairs, requiring nothing more in service than regularly scheduled inspections to ensure the longevity of the bondline. Many such repairs have been flying for decades with good results. As with anything involving adhesive bonding, proper surface preparation, and cleanliness of the bonding surfaces is crucial, while detailed and thorough training for the repair technicians is necessary. While these repairs are not difficult once the processes are understood, there are many small details, that can make the difference between a repair that will fail the first time it is loaded, and one that will last the lifetime of the structure.
Keeping up with the complexities of evolving materials, processes, and techniques of composite repair is challenging. However, the value of being able to design and execute high-quality repairs spurs technicians and engineers alike to continually learn and practice new repair solutions.
Photos Credit: Abaris Training Resources Inc.
Abaris Training Resources Inc.
Reno, NV
www.abaris.com
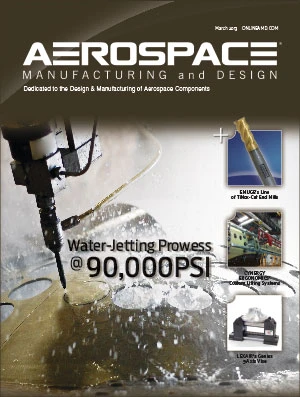
Explore the March April 2013 Issue
Check out more from this issue and find your next story to read.
Latest from Aerospace Manufacturing and Design
- NASA offers free high school engineering program this summer
- Pemamak's PEMA Vision 3D software
- Aerospace Industry Outlook - Spring 2025, presented by Richard Aboulafia
- Panama’s National Air and Naval Service selects Embraer Super Tucano
- Model 5770 Linear Abraser
- #42 Lunch + Learn Podcast - Quell Corp
- RECARO Aircraft Seating showcases advanced comfort solutions at AIX 2025
- Mitutoyo America’s MiSTAR 575 Shop Floor CNC CMM