Noise is one of the most serious constraints on expanding and improving the international air transport system. Greater air traffic and larger population growth in areas surrounding airports are increasing the impact of aircraft noise on the community.
Turbofan engine fan noise is one of the largest noise contributors, along with jet noise radiated by aircraft at take-off. The fan generates noise at its inlet similar to the noise caused by a propeller. The air swirls as it exits the fan. The swirling causes a loss of momentum, so the air is straightened out by running it through a set of stationary vanes called stators at the fan outlet to maintain momentum. The impact of the air on the stator blades is another major source of noise.
The acoustic liners that are built into the engine nacelle are fundamental to controlling fan noise. Acoustic liners present a major design challenge because they must address a large number of conflicting design requirements. Figure 1 shows the interior design of an engine with the location of the nacelles and acoustic liners.
Liners must provide high levels of noise reduction over a wide range of engine operating conditions and frequencies. Liners must also meet tight space restrictions and need to be as light as possible in order to limit fuel consumption. The liner is typically designed at a point when aspects of the airframe and engine are not completely defined, so the liner design must be flexible enough to adapt to changes. The liner must be able to survive exposure to heat, cold, water, oil, and maintenance operations. Finally, the liner must be durable enough to deliver decades of service in the highly demanding aircraft engine environment.
Improving acoustic liner design
Originally, the design of acoustic liners was based on static tests performed by running the engine on a test rig and measuring the radiated noise with an array of microphones. This approach was very expensive and designs could not be evaluated until a prototype engine was available for testing.
To address this challenge, Airbus engineers used analytical tools that predicted noise radiation. However, these tools were only accurate when used with very simple geometries. They were of limited usefulness in modeling noise radiation from the nacelle.
Airbus has long been working to simulate noise radiation from the nacelle more accurately. In 1999, the company joined a consortium developing more comprehensive acoustic simulation software called Actran. Since then, acoustic simulations have become a central element in Airbus’ noise control engineering.
“We are currently using it for design of nacelle liners in engine inlets and exhaust ducts, cockpit and cabin interior noise, air systems at ground, propellers, and contra-rotating propellers,” says Jean-Yves Suratteau, head of numerical methods in the Acoustics & Environment Department at Airbus.
The process is presented in Figure 1. The shape, or wetted surface, of the nacelle is defined by the aerospace engineers based on aerodynamic requirements. It is provided to acoustic engineers as a CAD file.
Engineers generate a finite element mesh of the nacelle liner geometry using hexahedral and tetrahedral elements. They check the quality of the mesh with a visualization tool. The model is based on a partitioned approach in which the inner acoustic domain is modeled with finite elements and the outer acoustic domain, which extends to infinity, is modeled using infinite elements. The acoustic source at the source plane is defined as a modal boundary condition. The incident acoustic field is defined in terms of rigid-wall duct modes.
The airflow inside and outside the engine has a major impact on acoustic radiation. An important preliminary step is to get computational fluid dynamics (CFD) results from the aerodynamicist engineers from Airbus. Engineers then use interpolated CFD temperature, velocity, and pressure data with the acoustic simulation.
Another input data for acoustic simulation are noise at fan levels, which is given by the engine manufacturers in the form of a modal decomposition obtained through engine static test results. A typical nacelle liner is composed of a sandwich constructed from a rigid back plate, honeycomb cells, and a perforated face sheet (single degree of freedom, SDOF). Among the key design parameters that typically can be changed to optimize the acoustic mesh of fan intake are the thickness of the liner, the spacing and diameter of holes in the face sheet, and the number of blocks of material from which the liner is constructed. Figure 2 (below) depicts the acoustic mesh.
The acoustic simulation results are provided in the form of sound pressure levels at various points around the nacelle as they would be measured by an array of microphones. The results can easily be correlated with static tests. Airbus has developed an in-house program that converts the results to effective perceived noise in decibels (EPNdB), the basic measurement for noise certification criteria. Figure 3 (below) depicts the results of an acoustic simulation at various points around the nacelle.
Liners are typically manufactured in two or three curved segments that are assembled with longitudinal splices. Simulation and other numerical tools helped to reveal the substantial impact of splices on forward fan noise. These simulations were confirmed with physical testing. These simulations made it possible to compute the radiated noise fields under all relevant engine-operating conditions and predict the noise reduction in certification conditions. The design of the zero-splice concept, through numerical simulation, made it possible to reduce fan noise and acoustic discomfort significantly.
Avoiding expensive design changes
Airbus developed an integrated numerical chain for Actran to make the software usable by acoustics engineers who are not numerical experts. The chain, called Automated Liner Optimization Chain for Nacelles Air Inlets and Exhausts (ANaNax), automates the simulation process from engine geometry to results, including prompting the user for all required information and performing validation checks on the data entered by users.
“A typical optimization loop for the nacelle liner requires evaluation of 80 liner iterations and three flight conditions at a frequency range from 125Hz to 5,650Hz. That means we need to simulate several thousand different cases,” Suratteau explains. “Robustness and accuracy of the simulations is critical, so realistic 3D shapes, flows, and boundary conditions are a must.”
Since, 2011, ANaNax has been used for six nacelle optimization studies in 2011 and eight in 2012. It is now the standard platform for nacelle design at Airbus, helping the company design and deliver acoustic solutions that save aircraft weight with a huge financial impact for airlines operating Airbus aircraft.
“Actran helps Airbus reduce product development costs by avoiding expensive post-design changes,” Suratteau says. “Having proven the value of Actran for nacelle liner design, Airbus is now in the process of expanding its use by developing new applications such as auxiliary power units (APUs) and ground systems for ramp noise, counter-rotating open rotor (CROR) engines for community noise, and cockpit and cabin interior noise.”
MSC Software
www.mscsoftware.com
About the author: Raphael Pesseau is general manager of Free Field Technologies (an MSC Software company).
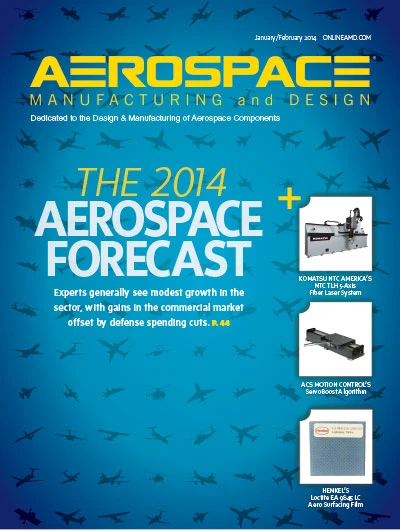
Explore the January February 2014 Issue
Check out more from this issue and find your next story to read.
Latest from Aerospace Manufacturing and Design
- US Dept. of Labor recognizes United Grinding as Apprenticeship Ambassador
- Optimal Engineering Systems' goniometers
- Breaking Free of the Additive Manufacturing Echo Chamber
- Don’t miss this month’s Manufacturing Lunch + Learn!
- marcus evans Aerospace & Defense Manufacturing and R&D Summit 2024
- How robots and cobots can work for you
- Why expertise in process fluid solutions is key to aerospace manufacturing performance
- Electra.aero to lead sustainable commercial airliner development